Atom

An atom is an extremely small piece of matter. All normal matter – everything that has mass – is made of atoms. This includes solids, liquids, and gases. The atom cannot be broken to parts by chemistry, so people once thought it was the smallest piece of matter that could exist.[1] There are over 100 different kinds of atoms, called chemical elements. Each kind has the same basic structure, but a different number of parts.
Atoms are very small, but their exact size depends on the type. Atoms are from 0.1 to 0.5 nanometers across.[2] One nanometer is about 100,000 times smaller than the width of a human hair.[3] This makes one atom impossible to see without special tools. Scientists learn how they work by doing experiments.
Atoms are made of three kinds of subatomic particles. These are protons, neutrons, and electrons. Protons and neutrons have much more mass. These are in the middle of the atom, called the nucleus. Lightweight electrons move quickly around them. The electromagnetic force holds the nucleus and electrons together.
Atoms with the same number of protons belong to the same chemical element. Examples of elements are carbon and gold. Atoms with the same number of protons, but different numbers of neutrons, are called isotopes. Usually an atom has the same number of electrons as protons. If an atom has more or less electrons than protons, it is called an ion, and has an electric charge.
Atoms can join by chemical bonds. Many things are made of more than one kind of atom. These are chemical compounds or mixtures. A group of atoms connected by chemical bonds is called a molecule. For example, a water molecule is made of two hydrogen atoms and one oxygen atom. The forming or breaking of bonds is a chemical reaction.
Atoms split if the forces inside are too weak to hold them together. This is what causes radioactivity. Atoms can also join to make larger atoms at very high temperatures, such as inside a star. These changes are studied in nuclear physics. Most atoms on Earth are not radioactive. They are rarely made, destroyed, or changed into another kind of atom.
History[change | change source]
The word "atom" comes from the Greek (ἀτόμος) "atomos", which means indivisible or uncuttable.[4] One of the first people to use the word "atom" is the Greek philosopher Democritus, around 400 BC. He thought that everything was made of particles called atoms, which could not be divided into smaller pieces. Some Hindu, Jain, and Buddhist philosophers also had ideas like this.[5] Atomic theory was a mostly philosophical subject, with not much scientific investigation or study, until the early 1800s.[6]
In 1777 French chemist Antoine Lavoisier defined the term element as we now use it. He said that an element was any substance that could not be broken down into other substances by the methods of chemistry. Any substance which could be broken down was a compound.[7]

In 1803, English philosopher John Dalton suggested that elements were made of tiny, solid balls called atoms. Dalton believed that all atoms of the same element have the same mass. He said that compounds are formed when atoms of more than one element combine. In any one compound, the atoms would always combine in the same numbers.[6][8]
In 1827, British scientist Robert Brown looked at pollen grains in water under his microscope. The pollen grains appeared to be shaking.[9] Brown used Dalton's atomic theory to describe patterns in how they moved. This was called Brownian motion. In 1905 Albert Einstein used mathematics to prove that the pollen particles were being moved by the motion, or heat, of individual water molecules. By doing this, he proved that atoms are real without question.[10][11]
In 1869, Russian scientist Dmitri Mendeleev published the first periodic table. The periodic table groups elements by their atomic number (how many protons they have; this is usually the same as the number of electrons). Elements in the same column, or group, usually have similar qualities.[12] For example, helium, neon, argon, krypton, and xenon are all in the same column and are very similar. All these elements are gases that have no color or smell. Also, they cannot combine with other atoms to form compounds. Together they are known as noble gases.
The physicist J.J. Thomson was the first person to discover electrons. This happened while he was working with cathode rays in 1897. He learned they had a negative charge, and the rest of the atom had a positive charge. Thomson made the plum pudding model, which said that an atom was like plum pudding: the dried fruit (electrons) were stuck in a mass of pudding (having a positive charge).
In 1909, Ernest Rutherford used the Geiger–Marsden experiment to prove that most of an atom is in a very small space, the atomic nucleus. Rutherford took a photo plate and covered it with gold foil. He then shot alpha particles (made of two protons and two neutrons stuck together) at it. Many of the particles went through the gold foil, which proved that atoms are mostly empty space. Electrons are so small and fast-moving that they did not block the particles from going through. Rutherford later discovered protons in the nucleus.[13]

In 1913, Niels Bohr created the Bohr model. This model showed that electrons travel around the nucleus in fixed circular orbits. This was better than the Rutherford model, but it was still not completely true.[14]
In 1925, chemist Frederick Soddy discovered that some elements had more than one kind of atom, called isotopes. Soddy believed that each different isotope of an element has a different mass.[15] To prove this, chemist Francis William Aston built the mass spectrometer, which measures the mass of single atoms. Aston proved that Soddy was right. He also found that the mass of each atom is a whole number times the mass of the proton.[16] This meant that there must be some particles in the nucleus other than protons. In 1932, physicist James Chadwick shot alpha particles at beryllium atoms. He saw that a particle shot out of the beryllium atoms. This particle had no charge, but about the same mass as a proton. He named this particle the neutron.[17]
The best model so far comes from the Schrödinger equation. Schrödinger learned that the electrons exist in a cloud around the nucleus, called the electron cloud. In the electron cloud, it is impossible to know exactly where electrons are. The Schrödinger equation says where an electron is likely to be. This area is called the electron's orbital.[18]
In 1937, German chemist Otto Hahn became the first person to make nuclear fission in a laboratory. He discovered this by chance when shooting neutrons at a uranium atom, hoping to make a new isotope. However, instead of a new isotope, the uranium changed into a barium atom, a smaller atom than uranium. Hahn had "broken" the uranium atom. This was the world's first recorded nuclear fission reaction.[19] This discovery led to the creation of the atomic bomb and nuclear power, where fission happens over and over again, creating a chain reaction.
Later in the 20th century, physicists went deeper into the mysteries of the atom. Using particle accelerators, they discovered that protons and neutrons were made of other particles, called quarks.[20]
Structure and parts[change | change source]
Parts[change | change source]

An atom is made of three main particles: the proton, the neutron, and the electron. Protons and neutrons have nearly the same size and mass (about 1.7×10−24 grams). The mass of an electron is about 1800 times smaller (about 9.1×10−28 grams). Protons have a positive charge, electrons have a negative charge, and neutrons have no charge. Most atoms have no charge. The number of protons (positive) and electrons (negative) are the same, so the charges balance out to zero. However, ions have a different number of electrons than protons, so they have a positive or negative charge.[21][1]
Scientists believe that electrons are elementary particles: they are not made of any smaller pieces. Protons and neutrons are made of quarks of two kinds: up quarks and down quarks. A proton is made of two up quarks and one down quark, and a neutron is made of two down quarks and one up quark.[20]
Nucleus[change | change source]
The nucleus is in the middle of the atom. It is made of protons and neutrons. The nucleus makes up more than 99.9% of the mass of the atom. However, it is very small: about 1 femtometer (10−15 m) across, which is around 100,000 times smaller than the width of an atom, so it has a very high density. [22]
Usually in nature, two things with the same charge repel or shoot away from each other. So for a long time, scientists did not know how the positively charged protons in the nucleus stayed together. We now believe that the attraction between protons and neutrons comes from the strong nuclear force. This force also holds together the quarks that make up the protons and neutrons. Particles called mesons travel back and forth between protons and neutrons, and carry the force.[23][24]

The number of neutrons in relation to protons defines whether the nucleus stays together or goes through radioactive decay. When there are too many neutrons or protons, the atom tries to make the numbers smaller or more equal by removing the extra particles. It sends out radiation in the form of alpha, beta, or gamma decay.[25] Nuclei can also change in other ways. Nuclear fission is when the nucleus breaks into two smaller nuclei, releasing a lot of energy. This release of energy makes nuclear fission useful for making bombs, and electricity in the form of nuclear power.[26] The other way nuclei can change is through nuclear fusion, when two nuclei join or fuse to make a larger nucleus. This process requires very high amounts of energy to overcome the electric repulsion between the protons, as they have the same charge. Such high energies are most common in stars like our Sun, which fuses hydrogen for fuel. However, once fusion happens, far more energy is released, because some of the mass becomes energy.[27]
The energy needed to break a nucleus into protons and neutrons is called its nuclear binding energy. This energy can be converted to mass, as stated by Einstein's famous formula E = mc2. Medium-sized nuclei, such as iron-56 and nickel-62, have the highest binding energy per proton or neutron. They will probably not go through fission or fusion, because they cannot release energy in this way. Very small and very large atoms have low binding energy, so they are most willing to go through fission or fusion.[28]
Electrons[change | change source]
Electrons orbit, or travel around, the nucleus. They are called the atom's electron cloud. They are attracted to the nucleus because of the electromagnetic force. Electrons have a negative charge, and the nucleus always has a positive charge, so they attract each other.[29]
The Bohr model shows that some electrons are farther from the nucleus than others in different levels. These are called electron shells.[29] Only the electrons in the outer shell can make chemical bonds. The number of electrons in the outer shell determines whether the atom is stable or which atoms it will bond with in a chemical reaction. If an atom has only one shell, it needs two electrons to be complete. Otherwise, the outer shell needs eight electrons to be complete.[30]
The Bohr model is important because it has the idea of energy levels. The electrons in each shell have a certain amount of energy. Shells that are farther from the nucleus have more energy. When a small burst of energy called a photon hits an electron, the electron can jump into a higher-energy shell. This photon must carry exactly the right amount of energy to bring the electron to the new energy level. A photon is a burst of light, and the amount of energy determines the color of light. So each kind of atom will absorb certain colors of light, called the absorption spectrum. An electron can also send out, or emit, a photon, and fall into a lower energy shell. For the same reason, the atom will only send out certain colors of light, called the emission spectrum.[29]
The complete picture is more complicated. Unlike the Earth moving around the Sun, electrons do not move in a circle. We cannot know the exact place of an electron. We only know the probability, or chance, that it will be in any place. Each electron is part of an orbital, which describes where it is likely to be. No more than two electrons can be in one orbital; these two electrons have different spin.

For each shell, numbered 1, 2, 3, and so on, there may be a number of different orbitals. These have different shapes, or point in different directions. Each orbital can be described by its three quantum numbers. The principal quantum number is the electron shell number. The azimuthal quantum number is represented by a letter: s, p, d, or f. Depending on the principal and azimuthal quantum numbers, the electron can have more or less energy. There is also a magnetic quantum number, but it does not usually affect the energy level. As more electrons are added, they join orbitals in order from lowest to highest energy. This order starts as follows: 1s, 2s, 2p, 3s, 3p, 4s, 3d, 4p, 5s, 4d. For example, a chlorine atom has 17 electrons. So, it will have:
- 2 electrons in the 1s orbital
- 2 electrons in the 2s orbital
- 6 electrons in the 2p orbitals
- 2 electrons in the 3s orbital
- 5 electrons in the 3p orbitals
In other words, it has 2 electrons in the first shell, 8 in the second shell, and 7 in the third shell. [31]
Properties[change | change source]
Atomic number[change | change source]
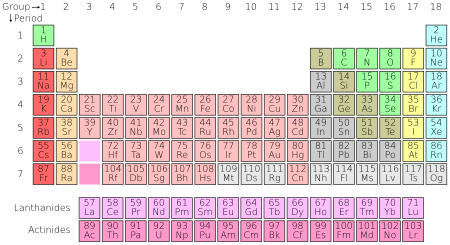
The number of protons in an atom is called its atomic number. Atoms of the same element have the same atomic number. For example, all carbon atoms have six protons, so the atomic number of carbon is six.[32] Today, 118 elements are known. Depending on how the number is counted, 90 to 94 elements exist naturally on earth. All elements above number 94 have only been made by humans.[33] These elements are organized on the periodic table.
Atomic mass and weight[change | change source]
Because protons and neutrons have nearly the same mass, and the mass of electrons is very small, we can call the number of protons and neutrons in an atom its mass number. Most elements have several isotopes with different mass numbers. To name an isotope, we use the name of the element, followed by its mass number. So an atom with six protons and seven neutrons is called carbon-13.
Sometimes, we need a more exact measurement. The exact mass of an atom is called its atomic mass. This is usually measured with the atomic mass unit (amu), also called the dalton. One amu is exactly 1/12 of the mass of a carbon-12 atom, which is 1.7×10−24 grams. Hydrogen-1 has a mass of about 1 amu. The heaviest atom known, oganesson, has a mass of about 294 amu, or 4.9×10−22 grams.[34] The average mass of all atoms of a particular element is called its atomic weight.[32]
Size[change | change source]
The size of an atom depends on the size of its electron cloud. Moving down the periodic table, more electron shells are added. As a result, atoms get bigger. Moving to the right on the periodic table, more protons are added to the nucleus. This more positive nucleus pulls electrons more strongly, so atoms get smaller.[35] The biggest atom is caesium, which is about 0.596 nanometers wide according to one model. The smallest atom is helium, which is about 0.062 nanometers wide.[36]
How atoms interact[change | change source]
When atoms are far apart, they attract each other. This attraction is stronger for some kinds of atoms than others. At the same time, the heat, or kinetic energy, of atoms makes them always move. If the attraction is strong enough, relative to the amount of heat, atoms will form a solid. If the attraction is weaker, they will form a liquid, and if it is even weaker, they will form a gas.

Chemical bonds are the strongest kinds of attraction between atoms. The movement of electrons explains all chemical bonds. Atoms usually bond with each other in a way that fills or empties their outer electron shell. The most reactive elements have an almost full or almost empty outer shell. Atoms with a full outer shell, called noble gases, do not usually form bonds.[38]
There are three main kinds of bonds: ionic bonds, covalent bonds, and metallic bonds.
- In an ionic bond, one atom gives electrons to another atom. Each atom becomes an ion: an atom or group of atoms with a positive or negative charge. The positive ion (which has lost electrons) is called a cation; it is usually a metal. The negative ion (which has gained electrons) is called an anion; it is usually a nonmetal. Ionic bonding usually results in a regular network, or crystal, of ions held together.
- In a covalent bond, two atoms share electrons. This usually happens when both atoms are nonmetals. Covalent bonds often form molecules, ranging in size from two atoms to many more. They can also form large networks, such as glass or graphite. The number of bonds that an atom makes (its valency) is usually the number of electrons needed to fill its outer electron shell.
- In a metallic bond, electrons travel freely between many metal atoms. Any number of atoms can bond this way. Metals conduct electric current because electric charge can easily flow through them. Atoms in metals can move past each other, so it is easy to bend, stretch, and change the shape of metals.[39]
All atoms attract each other by Van der Waals forces. These forces are weaker than chemical bonds. They are caused when electrons move to one side of an atom. This movement gives a negative charge to that side. It also gives a positive charge to the other side. When two atoms line up their sides with negative and positive charges, they will attract.[40]
Although atoms are mostly empty space, they cannot pass through each other. When two atoms are very close, their electron clouds will repel each other by the electromagnetic force.[41]
Magnetism[change | change source]
To understand how magnets work, we can look at the properties of the atom. Any magnet has a north and south pole, and a certain strength. The direction and strength of a magnet, together, are called its magnetic moment. Every electron also has a magnetic moment, like a tiny magnet. This comes from the electron's spin and its orbit around the nucleus. The magnetic moments for the electrons add up to a magnetic moment for the whole atom. This tells us how atoms act in a magnetic field.
Every electron has one of two opposite spins. We can think of one as turning to the right, and the other as turning to the left. If every electron is paired with an electron with the opposite spin in the same orbital, the magnetic moments will cancel out to zero. Atoms like this are called diamagnetic. They are only weakly repelled by a magnetic field.
However, if some electrons are not paired, the atom will have a lasting magnetic moment: it will be paramagnetic or ferromagnetic. When atoms are paramagnetic, the magnetic moment of each atom points in a random direction. They are weakly attracted to a magnetic field. When atoms are ferromagnetic, the magnetic moments of nearby atoms act on each other. They point in the same direction. This means that the whole object is a magnet, and it can point in the direction of a magnetic field. Ferromagnetic materials, such as iron, cobalt, and nickel, are strongly attracted to a magnetic field.[42]
Radioactive decay[change | change source]

Some elements, and many isotopes, have what is called an unstable nucleus. This means the nucleus is either too big to hold itself together, or it has too many protons or neutrons.[43] When a nucleus is unstable, it has to eliminate the excess mass of particles. It does this through radiation. An atom that does this is called radioactive. Unstable atoms emit radiation until they lose enough particles in the nucleus to become stable. All atoms above atomic number 82 (82 protons, lead) are radioactive.[44]

There are three main kinds of radioactive decay: alpha, beta, and gamma.[25]
- Alpha decay is when the atom shoots out a particle having two protons and two neutrons. This is a helium-4 nucleus. The result is an element with an atomic number of two less than before. So, for example, if a uranium atom (atomic number 92) went through alpha decay, it would become thorium (atomic number 90). Alpha decay happens when an atom is too big and needs to lose some mass.
- Beta decay is when a neutron turns into a proton, or a proton turns into a neutron. In the first case, the atom shoots out an electron. In the second case, it shoots out a positron (like an electron but with a positive charge). The result is an element with one higher or one lower atomic number than before. Beta decay happens when an atom has either too many protons or too many neutrons.
- Gamma decay is when an atom shoots out a gamma ray, or wave. It happens when there is a change in the energy of the nucleus. This is usually after a nucleus has gone through alpha or beta decay. There is no change in the atom's mass, or atomic number, only in the stored energy inside the nucleus, in the form of particle spin.
Every radioactive element or isotope has a half-life. This is how long it takes half of any sample of atoms of that type to decay into a different isotope or element.[45]
Creation of atoms[change | change source]
Nearly all the hydrogen atoms in the Universe, most of the helium atoms, and some of the lithium atoms were made soon after the Big Bang. Even today, about 90% of all atoms in the Universe are hydrogen.[46]
All other atoms come from nuclear fusion in stars, or sometimes from cosmic rays that hit atoms. At the start of their life, all stars fuse hydrogen to make helium. The least massive stars, red dwarfs, are expected to stop there. All other stars will then fuse helium to make carbon and oxygen. In stars like the Sun, the temperature and pressure are too low to make larger atoms. But more massive stars continue fusion, until they create iron (atomic number 26) or nickel (atomic number 28). [47] Atoms can also grow larger when neutrons or protons hit them. This could happen inside stars or in supernovae. Most atoms on Earth were made by a star that existed before the Sun.[48]
People make very large atoms by smashing together smaller atoms in particle accelerators. However, these atoms often decay very quickly. Oganesson (element 118) has a half-life of 0.00089 seconds. Even larger atoms may be created in the future.[34]
Related pages[change | change source]
- Atomic physics, for more detail about the physics of atoms
- Atomic theory, for more detail about the history
- Chemical element, each kind of atom
- Exotic atom, an atom with different parts instead of protons, neutrons, and electrons
- Quantum mechanics, the study of small particles and how they interact with energy
- States of matter, the different forms in which atoms or molecules can be found
Sources[change | change source]
References[change | change source]
- ↑ 1.0 1.1 "What is an atom ?". NRC Web. March 19, 2020. Retrieved December 6, 2022.
- ↑ Philip, Michael; Dong, Judy (1998). Elert, Glenn (ed.). "Size of an Atom". The Physics Factbook. Archived from the original on January 30, 2022.
- ↑ Ley, Brian (1999). Elert, Glenn (ed.). "Diameter of a Human Hair". The Physics Factbook. Archived from the original on July 11, 2022.
- ↑ "Atom Definition & Meaning". Dictionary.com. Retrieved November 28, 2022.
- ↑ American Chemical Society 2010, pp. 21–33.
- ↑ 6.0 6.1 American Chemical Society 2010, pp. 1–5.
- ↑ Chalmers 2009, p. 168.
- ↑ Chalmers 2009, pp. 177–179.
- ↑ Chalmers 2009, p. 234.
- ↑ Lee, Y.K.; Hoon, Kelvin. "Brownian motion - a history". Archived from the original on December 18, 2007. Retrieved November 30, 2009.
- ↑ Chalmers 2009, p. 239.
- ↑ Flowers et al. 2019, pp. 165–169.
- ↑ Flowers et al. 2019, pp. 73–79.
- ↑ Flowers et al. 2019, pp. 131–135.
- ↑ "Frederick Soddy – Biographical". NobelPrize.org. Nobel Prize Outreach AB. Retrieved August 22, 2022.
- ↑ "Francis W. Aston – Biographical". NobelPrize.org. Nobel Prize Outreach AB. Retrieved August 7, 2022.
- ↑ American Chemical Society 2010, pp. 65–81.
- ↑ Orchin, Milton; Macomber, Roger S.; Pinhas, Allan; Wilson, R. Marshall (2005). "Atomic Orbital Theory" (PDF). The Vocabulary and Concepts of Organic Chemistry (2nd ed.). John Wiley & Sons, Inc.
- ↑ "Otto Hahn, Lise Meitner and Fritz Strassman". Science History Institute. December 7, 2017. Retrieved August 22, 2022.
- ↑ 20.0 20.1 Riordan, Michael (1992). "The Discovery of Quarks". Science. 256 (5061): 1287–1293. Bibcode:1992Sci...256.1287R. doi:10.1126/science.256.5061.1287. ISSN 0036-8075. JSTOR 2877300. PMID 17736758. S2CID 34363851 – via JSTOR.
- ↑ Flowers et al. 2019, p. 80.
- ↑ Flowers et al. 2019, p. 79.
- ↑ "Nobel Prize in Physics 1949 – Presentation Speech". NobelPrize.org. Nobel Prize Outreach AB. Retrieved May 13, 2022.
- ↑ Aoki, Sinya; Hatsuda, Tetsuo; Ishii, Noriyoshi (January 2010). "Theoretical Foundation of the Nuclear Force in QCD and Its Applications to Central and Tensor Forces in Quenched Lattice QCD Simulations". Progress of Theoretical Physics. 123 (1): 89–128. arXiv:0909.5585. Bibcode:2010PThPh.123...89A. doi:10.1143/PTP.123.89. S2CID 18840133.
- ↑ 25.0 25.1 Flowers et al. 2019, pp. 1086–1088.
- ↑ Flowers et al. 2019, pp. 1100–1105.
- ↑ Flowers et al. 2019, pp. 1110–1111.
- ↑ Iliadis 2007, pp. 33–34.
- ↑ 29.0 29.1 29.2 Flowers et al. 2019, pp. 128–135.
- ↑ Flowers et al. 2019, p. 215.
- ↑ Flowers et al. 2019, pp. 148–153.
- ↑ 32.0 32.1 Flowers et al. 2019, pp. 79–85.
- ↑ McMahon, Mary (July 27, 2022). "How Many Elements on the Periodic Table of the Elements Occur Naturally?". All the Science. Retrieved August 22, 2022.
- ↑ 34.0 34.1 "Oganesson | Og (Element) - PubChem". pubchem.ncbi.nlm.nih.gov. Retrieved August 6, 2022.
- ↑ Flowers et al. 2019, pp. 158–160.
- ↑ Clementi, E.; Raimond, D. L.; Reinhardt, W. P. (1967). "Atomic Screening Constants from SCF Functions. II. Atoms with 37 to 86 Electrons". Journal of Chemical Physics. 47 (4): 1300–1307. Bibcode:1967JChPh..47.1300C. doi:10.1063/1.1712084.
- ↑ Chung, D. D. L. (2002). "Review Graphite". Journal of Materials Science. 37 (8): 1475–1489. doi:10.1023/A:1014915307738. S2CID 189839788.
- ↑ Reusch, William (July 16, 2007). "Virtual Textbook of Organic Chemistry". Michigan State University. Archived from the original on October 21, 2007.
- ↑ "Fundamentals of Chemical Bonding". LibreTexts. August 15, 2020. Retrieved May 18, 2022.
- ↑ Swinerd, Vicky (2003). "What are Van der Waals Forces?".
- ↑ Frank, Adam (April 7, 2015). "Why Doesn't Your Butt Fall Through The Chair?". NPR.
- ↑ Serway, Moses & Moyer 1997, pp. 476–484.
- ↑ Serway, Moses & Moyer 1997, pp. 533–534.
- ↑ Sills, Alan D. (2003). Earth Science the Easy Way. Barron's Educational Series. pp. 131–134. ISBN 978-0-7641-2146-3. OCLC 51543743.
- ↑ Flowers et al. 2019, p. 1090.
- ↑ Grochala, Wojciech (March 2015). "First there was hydrogen". Nature Chemistry. 7 (3): 264. Bibcode:2015NatCh...7..264G. doi:10.1038/nchem.2186. ISSN 1755-4349. PMID 25698337.
- ↑ Iliadis 2007, pp. 11–27.
- ↑ Iliadis 2007, pp. 568–570.
Bibliography[change | change source]
- Chalmers, Alan (2009). The scientist's atom and the philosopher's stone : how science succeeded and philosophy failed to gain knowledge of atoms. [Dordrecht]: Springer. ISBN 978-90-481-2362-9. OCLC 432702848.
- Flowers, Paul; Theopold, Klaus; Langley, Richard; Neth, Edward J.; Robinson, William R. (2019). Chemistry: Atoms First (2nd ed.). Houston, Texas: OpenStax, Rice University. ISBN 978-1-947172-63-0. OCLC 1089692119.
- Giunta, Carmen, ed. (2010). Atoms in chemistry : from Dalton's predecessors to complex atoms and beyond. Washington, DC: American Chemical Society. ISBN 978-0-8412-2558-9. OCLC 659536310.
- Iliadis, Christian (2007). Nuclear Physics of Stars. Weinheim: Wiley-VCH. doi:10.1002/9783527618750. ISBN 978-3-527-40602-9.
- Serway, Raymond A.; Moses, Clement; Moyer, Curt A. (1997). Modern Physics (2nd ed.). Saunders College Publishing. ISBN 0-03-001547-2.
Other websites[change | change source]



- Atom (science) -Citizendium
- General information on atomic structure Archived December 23, 2012, at the Wayback Machine
- "A Brief History of the Atom". Archived from the original on December 9, 2009. Retrieved November 30, 2009.
- "Structure of Atom: Class 11 Chemistry NCERT Chapter 2". Reeii Education. May 30, 2020. Archived from the original on October 22, 2020. Retrieved October 18, 2020.
- "How does radioactive decay work?".
- "Atomic Emission Spectra - Origin of Spectral Lines". Archived from the original on February 28, 2006. Retrieved May 2, 2022.
- "S-Cool: Types of radiation".